Industry has for many years relied on nonrenewable resources as cathodic protection materials. An alternative approach is discussed for the built environment where repurposed industrial wastes produce alkali-activated cementitious or geopolymer conductive anode binders. These hardened mortars and concrete activate galvanic anodes for net-zero reinforced concrete repair as well as sustainable impressed current anodes sprayed, chased into decks, or tucked within mortar joints.
Case studies are highlighted in the United States and United Kingdom that demonstrate success in different structures and exposure conditions. These systems are also installed with imbedded corrosion rate monitoring to allow remote service life tracking of performance.
As the world responds to the climate crisis with intent to control emissions and effect changes to ensure global temperature rises remain below 1.5 °C, industry and investments are focusing on technology and innovations to also respond to the challenge.
The corrosion and protection industry has its own challenges toward decarbonization given the tendency for business to maintain fossil fuel industries and use technologies that are largely nonrenewable.
Examples of this are seen in cathodic protection (CP) with the use of sacrificial anodes that once they corrode, cannot be recycled and therefore, their embodied carbon is lost forever. This is true for oil and gas as well as concrete applications. Moreover, with impressed current CP (ICCP) anodes and the use of rare earth metals to form dimensionally stable oxides on titanium substrates that in turn are embedded (for concrete) cannot easily be repurposed.
These anodes do have longevity in use, however, and use of ICCP is reported to be the best option to preserve embodied carbon1 in reinforced concrete structures. Competition with other industries for such metals and their impact on the environment in their extraction are likely to come under increasing scrutiny.
Cement is an essential industry but with Portland cement producing around 8% of harmful global emissions, this is not surprisingly receiving focus with research and development of alternatives and adjusted Portland formulations to drive down such emissions.
This article discusses an innovative approach taken for applications in the built environment to repurpose industrial wastes as an alternative alkali-activated (or geopolymer) cementitious material (AACM) that can not only be used to repair and build concrete structures but has been developed in the form of an anode AACM binder that activates galvanic anodes and can act as an ICCP anode in mortar and concrete.
Development and Impact of Sustainable Materials
AACMs are identified2 as a positive source of change to the cement industry as these are produced with no heat (ambient blending) from waste streams arising from fossil fuel, steel, and mining and when incorporated in mix designs form a hardened material that requires reduced water content.
This contributes to a carbon dioxide (CO2) emissions reduction calculated to be 55 kg/tonne vs. 900 kg/tonne or over 90% when compared to ordinary Portland cement (OPC).
Figures 1 and 2 show the aged compressive and flexural strengths that are comparable with Portland concretes and mortars with good early and mature strength gains from standard prism tests. Other improved characteristics include no shrinkage, high resistance to fire (>1,200 °C for 5 h), chemicals (acids, sulfates, phosphates), and alkali-silica reaction raising hopes that these AACMs have a future as an alternative binder in many structures and exposure conditions.
Arguably, the most useful derivative is the ability of these materials to act as the anode and decarbonize CP for concrete and masonry structures. Formulations have been developed and put into practice that, in the laboratory, show protection to 128 mA/m2 of steel surface area before acid generation forms.
This has allowed confidence for technical design grading at much lower anode current densities presently used for concrete applications, typically 10 mA/m2 at the steel such that acid cannot generate. Confidence is further gained by the high acid resistance offered by AACMs that underpins their effectiveness.
The high alkalinity (pH 14) of the wet and hardened material is used to activate and maintain activation of zinc for use in concrete repairs to protect incipient (ring) anode effects from forming around the perimeter of the patch. This acts as the macro-cathode, from when the fresh repair mortar is placed, and the perimeter steel outside the patch balances the redox reaction.
The dimensionally stable nature of the material and its controllability allows its use as an ICCP anode. It has the ability to form as a mortar, grout, or concrete conventionally placed by cast in-place or cut and grouted into decks to incorporate into existing reinforced concrete structures, such as parking and civil infrastructure, as well as gunned into joints for transitional steel frame masonry-clad buildings.
This has a positive impact to climate change, yielding a high embodied saving of carbon that contributes significantly toward net zero and an owner’s environment social governance compliance.
Embodied carbon within buildings has been estimated3 to be 19,180 tonnes of CO2e higher if demolished than if it were preserved and restored.
By utilizing sustainable anode materials to control existing building stock, these savings replicate across owner portfolios to offer gigatons of CO2 emission savings globally. When coupled with AMPP’s assessment of corrosion problems at 70% of infrastructure affected and consequential high savings4 of >$800 m per annum when control technologies are adopted, then these offer major sustainability contributions on all measures.
The use of online control and reporting of data from embedded corrosion rate probes as part of the ICCP designed system also provides the platform to inform on service life by tracking performance over time5 that gives owners tangible and accountable records of the benefits of adopting such technology within their structure (Figure 3).
Use for Existing Reinforced Concrete Structures
These innovations are put to good use at an underground parking facility in Edinburgh, Scotland, where an interoperable corrosion management design, following a comprehensive condition assessment of the parking decks, saw the targeted use of specific technologies aligned with risk gleaned from the assessment to design the best economic mix of technologies to achieve the enhanced service life expectation of 25 extra years of service (Figure 4, top).
Of the four decks protected, access ramps and Levels -2, and half of -3 underwent the use of the ICCP anode material chased into the decks associated with the support beams and throwing current into the 600-mm gap between chase lines. In addition, an area of structural beam and wall was protected using a sprayed concrete version of the ICCP anode installation.
The other half of Level -3 and all of Levels -1 and -4 used bare zinc anodes tied to the steel reinforcement within the perimeter of the concrete repairs and were activated by use of the conductive AACM packing mortar before placing the concrete repair material.
The remaining areas to Levels -1, -3, and -4 not protected locally by the galvanic anodes were protected using surface-applied corrosion inhibitor before all levels were further protected with a waterproofing membrane for function and aesthetic.
The whole structure is protected in 44 controllable zones with an additional seven zones for monitoring inhibitor performance to assess replenishment timing.
Use in Masonry-Clad Transitional Steel Frame Buildings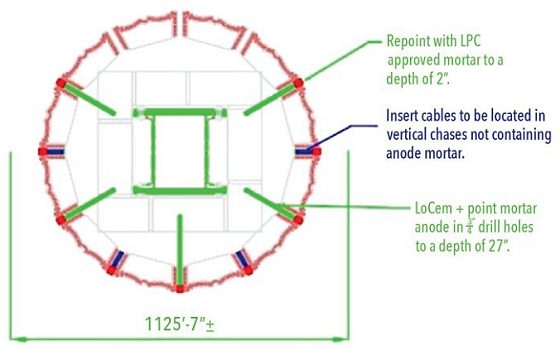
Transitional steel frame buildings date largely between 1900-1950 and are present in most developed cities when the drive to taller buildings meant that load-bearing masonry could no longer be the structural design of choice and steel beams and stanchions were necessary.
Cladding with stone and/or brick and infilling with mason’s mortar inadvertently provided a means to trap moisture that over the decades results in the initiation and propagation of corrosion with the resultant build-up of exfoliated corrosion products. These exert pressure on the masonry, causing movement, cracking, and ultimately failure with collapse.
In New York City, the technology was adopted in a targeted way to protect the colonnade steel behind terracotta at Levels 29 to 32 (see Figure 5 demonstrating anode placement in sectional detail) at a well-known prestigious property on Park Avenue.
The modularity of the power, control, and monitoring system allows expansion on the installed open network for other parts of the property in the future (Figure 6).
Use in New Structures
Undoubtedly the most effective moment to incorporate ICCP is at the construction stage, in so-called cathodic prevention preservation, where the anode can be placed prior to the concrete being applied either in-situ or in the precasting factory.
The use of this sustainable anode mortar in molded componentized form allows a zonal array of modular anode units to be attached directly to the steel reinforcement during the steel fabrication stage spaced by plastic connections to de-risk the possibility of short-circuiting. The anode array is interconnected by a plug-and-play modular wiring loom and terminated in a networked power, control, and monitoring system before commissioning and operation online. Concrete is then placed in-situ or within precast factories to manage quality of the structural element.
Research6 has shown that such designs can be optimized to provide certainty to resilience and long service life without replacement.
Summary
Forming CP anodes as a build material from sustainable origins has a significant impact toward net zero targets by repurposing industrial wastes, preserving embodied carbon for the structure’s whole life, and providing sustainability with future proofing.
It was described here with some working systems that this can be a successful strategy as a positive legacy for the next generation to avoid the disruption and costs associated with deterioration of buildings and infrastructure without dependency on the negative impact of nonrenewable material resources.
Online remote control and monitoring also provide a running record of performance without the need for travel impacts on the environment.
Acknowledgements
Our thanks and appreciation go to Professors Mangat and Lambert at Sheffield Hallam University and Mott MacDonald for their in-depth knowledge of this technology and their support throughout its development, and to all the engineers, contractors, and owners who trusted these innovations to protect their assets into the future.
References
1 C. Atkins, P. Lambert, “Sustainability and Corrosion,” Materials & Corrosion (November 2021).
2 G. Jones, P. Lambert, “Characterisation of Low-Carbon AACM Concrete and Mortar,” Concrete 50, 9 (2016): pp. 50-52.
3 P. Oldfield, “Retrofit First,” The Architects Journal (October 2021).
4 “International Measures of Prevention, Application and Economics of Corrosion Technologies Study” (Houston, TX: NACE International, 2016).
5 G. Jones, P. Lambert, “Predicting Service Life From Site-Accessed Corrosion Rate Data,” Forensic Engineering 169, 2 (2016).
6 F. O-Flaherty et al., “Optimizing Cathodic Protection Design for Maximum Bond Performance in Reinforced Concrete,” Materials & Corrosion 69, 9 (2018): pp. 1151-1162.