From aircraft and ships to ground vehicles, buildings, and infrastructure, the U.S. Department of Defense (DoD) acquires, operates, and maintains a vast array of physical assets to perform its mission of providing the military forces needed to deter war and to protect the security of the United States. While implementing this mission, the DoD must train and fight in some of the most corrosively aggressive environments on Earth. As a result, DoD assets are vulnerable to significant degradation due to corrosion, with corrosion costs estimated to be between $10 billion and $20 billion annually.1
For the DoD’s fleet of aircraft, environmental exposure can cause corrosion damage that leads to degradation of the airframe structure, electrical wiring and interconnection system, and avionics, says Jeff Demo, electrical engineer with Luna Innovations, Inc. (Charlottesville, Virginia). Using fiscal year (FY) 2007 and 2008 as a measurement baseline, the estimated annual cost of corrosion for U.S. Navy and Marine Corps aviation is $3 billion, or 31.5% of maintenance costs. Using FY 2008 and 2009 as a baseline, the estimated annual corrosion cost for U.S. Air Force aviation and missiles is $4.5 billion, or 24% of maintenance costs. As an example, corrosion has been identified as the reason for more than 50% of the maintenance needed on the Boeing KC-135 Stratotanker. Additionally, corrosion is a contributing factor in ~2.1 million hours of non-availability for all Air Force aviation assets, which equates to an average of 15.9 days of corrosion-related non-availability per year for every aircraft in active status.1-2
A leading cost driver is the inspection time used to evaluate an aircraft for corrosion, Demo says. While nondestructive evaluation (NDE) techniques such as ultrasonic testing have been developed for aircraft inspection, the primary means used by military maintainers to assess an aircraft for corrosion is generally a schedule-based inspection of the airframe conducted manually by maintenance personnel, which is labor intensive, requires aircraft downtime, and may not reveal damage until substantial repairs are necessary. Corrosion damage that has occurred in readily accessible areas is identified by these inspections, he notes; however, many times corrosion damage or its precursors can go undetected and untreated in locations that are difficult to access, which can intensify structural damage, cause safety issues, and lead to more extensive repairs or component replacement that cause maintenance costs to escalate.
One of the biggest challenges encountered when conducting an airframe inspection is reaching blocked, sealed, or obstructed areas, Demo adds. “In order to do a full comprehensive inspection, there is a lot of upfront work that has to be done to access these areas for inspection,” he says. Airframe assessment typically requires maintenance personnel to break seals, open panels, remove floorboards, etc. so that inaccessible areas can be reached and visual observations and evaluations can be done. A potential corrosion problem can still be missed, though, because it isn’t visible—it may be under a coating, or the nature of the corrosion makes it difficult to detect with the human eye. Also, Demo says, repeatedly opening panels and breaking seals designed into the aircraft can increase exposure of the airframe’s vulnerable areas to environmental threats such as humidity and chlorides. “At that point, instead of preventing corrosion by doing these inspections, you can potentially cause more corrosion,” he adds.
Airframe Corrosion
Airframes, which are primarily constructed of aluminum alloys, are susceptible to several types of localized corrosion, which frequently occurs without any outward signs of damage, Demo comments. Localized corrosion can include general surface corrosion of the airframe due to atmospheric exposure; pitting corrosion, which can weaken the structure and lead to cracking; crevice corrosion that forms in gaps or areas where there is contact between parts; aluminum exfoliation, a form of intergranular corrosion where the corrosion products have more volume than the parent metal and the increased volume forces the metal to separate into layers and delaminate; stress corrosion cracking (SCC), where a crack will propagate under tensile stress in a corrosive environment, which can lead to a sudden, unexpected failure; and corrosion fatigue, which is mechanical degradation of the metal when it is subjected to both cyclic loading and corrosion. Because fasteners used in aircraft are often comprised of steel and other non-aluminum alloys, Demo adds, airframes also can be degraded by galvanic corrosion, which occurs when two dissimilar metals in contact with each other drive the corrosion process when an electrolyte is present.
Generally, conditions such as temperature, humidity, precipitation, and the presence of atmospheric chemicals such as chlorides will affect the corrosiveness of an environment, and these conditions can vary in different compartments or areas within a single airframe, which Demo refers to as microclimates. ISO standards 92233 and 92244 use atmospheric parameters under specific conditions to predict the severity of the corrosive environment (from C1 for very low corrosivity to C5 for very high corrosivity). They also provide guidelines to estimate the corrosion rates of steel, zinc, copper, and aluminum using time of wetness (TOW), which is based on the amount of time the relative humidity (RH) is above 80%; the sulfur dioxide (SO2) deposition rate; and airborne salinity.
Corrosion Monitoring System
Demo comments that inspection and maintenance activities, as well as aircraft safety and operational availability, could be significantly enhanced for both fixed and rotary wing aircraft exposed to corrosive environments by using a corrosion monitoring system that identifies environmental conditions that lead to corrosion and also detects corrosion damage. The monitoring system would measure corrosivity parameters in microclimates within an airframe, and the resulting data could be analyzed to evaluate the degree of environmental severity for those areas based on ISO 9223 classifications and estimate the probability that an area is experiencing corrosion that requires inspection or maintenance actions.
As part of a Small Business Innovation Research (SBIR) program for the Naval Air Systems Command (NAVAIR), Luna Innovations developed a self-contained multi-modal corrosion sensor system, LS2A†, to measure, record, and analyze environmental and corrosivity parameters that affect corrosion susceptibility and corrosion rate of a material.5 This corrosion monitoring system comprises a set of sensors combined in a small node (3 by 2.4 by 1.4 in [76 by 61 by 36 mm]) that measures relative humidity (RH), air and surface temperature, solution conductivity (i.e., the resistance of any condensate on the sensor), and the corrosion rate of aluminum, as well as a smart transducer interface module with multiple transducers. The sensor technologies are integrated with an embedded low-power, wireless electronics package that collects and processes measurements from the sensors and reports both raw sensor measurement data and an ISO 9223-based environmental severity classification (C1 to C5) based on the sensor output. An integrated real-time clock generates time stamps of all monitored events, and an onboard flash memory stores data for extended time periods. Data can be downloaded via a wireless link or a standard USB to RS-485 data transfer cable to a computer program that provides the user with a graphical interpretation of the data. The sensor nodes were validated at ground-based exposure sites through long-term exposure testing in conjunction with bare aluminum coupons and aluminum coupons joined with dissimilar metal fasteners. The coupons were analyzed for material loss when real-time sensor data were collected, and the results from both correlated with realistic matches.
Demo comments that military aircraft typically have a long history of manual inspections and their maintainers generally have identified areas on each airframe that are known corrosion “hotspots,” such as underneath a helicopter’s floorboards or on its tail boom. Some of these areas, he adds, are difficult to access and inspect. A sensor node placed within these hotspot areas can provide the structure’s corrosivity information and corrosion rates to maintainers, which enables them to schedule manual inspections and maintenance actions based on the predicted condition of the structures. If the data indicate that the structure is in one of the ISO 9223 edge conditions (e.g., a C5), ultimately that may drive an immediate action because there is a potentially severe corrosion issue that can’t wait for the next scheduled manual inspection. In the case of a C1 condition, he says, no action may be necessary until the maintenance schedule calls for an inspection because little or no corrosion activity is indicated.
A number of sensor nodes distributed at various corrosion hotspots throughout an airframe provides a view of the overall structural health of the aircraft as well as an assessment of the structural integrity within individual microclimates, which gives maintainers a method for tracking and anticipating the corrosive effect of various geographic environments on the entire aircraft as well as individual structures. Because the sensor system is capable of measuring, processing, and storing instantaneous corrosion rates as well as long-term cumulative corrosion data, current real-time corrosion information along with the ongoing corrosion history of a particular structure being monitored is available to the maintainer. This provides a record of corrosion levels between inspections, which may help maintainers pinpoint short-term events that correspond with particularly high corrosion rates occurring during the interim and develop mitigation strategies to address them.
During a five-month testing period at the Patuxent River Naval Air Station (Patuxent River, Maryland), a wired and wireless corrosion monitoring sensor node were installed in the avionics bay on the Bell UH-1N Twin Huey T-Rex† rotorcraft test bed at the Naval Aviation Center for Rotorcraft Advancement (NACRA).6 To determine how the conditions inside the airframe compared with external environmental conditions, data were periodically collected from the sensor nodes inside the test body throughout the testing period and correlated with the ambient environmental conditions reported in the same time frame by the naval air station’s weather station. Demo comments that results of RH measurements from the ambient environment and inside the test body were not the same—the RH measurements inside the airframe were generally significantly lower than for ambient conditions.
“This shows us that knowing the relative humidity outside of the aircraft doesn’t necessarily tell us what is going on inside the airframe,” he says. However, he adds, when the airframe’s actual RH measurements were analyzed along with its corresponding surface and air temperatures to determine the effective RH, the results correlated well with ambient air measurements. “The effective relative humidity provides an estimate of what the actual relative humidity should be inside the airframe based on the external environmental conditions,” he explains. “If there is a relative humidity measurement within the aircraft that is significantly different from what our effective relative humidity tells us it should be, then we know there is a situation where something other than ambient conditions is affecting what is going on inside the airframe.”
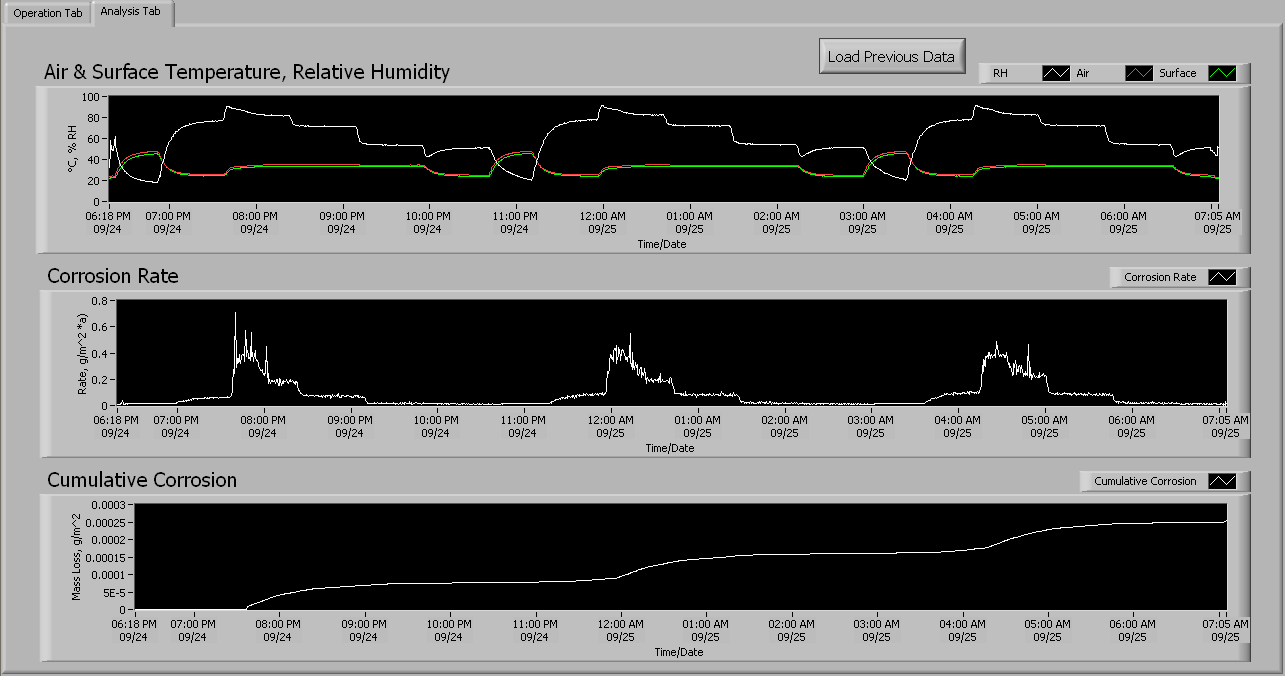
The test rotorcraft results also showed a strong seasonal trend in the data collected for all conditions, Demo notes. In addition to reduced RH measurements inside the airframe during winter months, a reduction in condensate conductivity (which indicates a reduction in the amount of chlorides present in the environment) and corrosion rates were seen during this time period as well. The close correlation between seasons and corrosivity readings may provide additional information to maintainers that could lead to a reduced frequency of inspections during winter months.
Knowing how environmental parameters affect the corrosion susceptibility and corrosion rate of aluminum will help aircraft maintainers to schedule maintenance more efficiently, which can improve overall fleet safety, increase aircraft availability, and reduce operational costs.
†Trade name.
References
1 “Why DoD Must Protect Its Assets,” CorrDefense, Director’s Corner, https://www.corrdefense.org/External/WhyDoDMustProtectItsAssets.aspx (December 3, 2014).
2 E. Herzberg, “DoD Releases a Report on the Effects of Corrosion on the Cost and Availability of Air Force Aircraft and Missiles,” CorrDefense 8, 1 (2012).
3 ISO 9223:2012, “Corrosion of metals and alloys—Corrosivity of atmospheres—Classification, determination and estimation” (Geneva, Switzerland: ISO, 2012).